Sugar High
Project Background:
How do things that spew fire and fly at hundreds of miles per hour not get anyone's attention. But more recently, SpaceX and their attempt to bring down 10 story high rocket stages weighing 4 tons on autonomous sea fairing landing platforms did the trick for me to try and make my own flying flame farters.
Project Plan:
Watch some rocket launches on You-Tube
Build a rocket!
Goal of contraption:
-
Get a thing as high as possible only using materials avaible to me in my immediate vicinity.
-
Do so without posing a risk to anything other than the thing being launched.
-
Be known as the rocket guy
-
If above mentioned goal is not reached, proceed to call myself the rocket guy.
Single most basic applied principle:
-
Things that push get pushed.
I guess we need to quantify that. To push a thing, the difficulty in pushing it depends on how heavy it is and how fast you pushed it.
If you push something, its pushedness is how heavy it is and how fast it goes. Some people call this momentum. Anyways,
since relativity is impartial (and I mean, it seriously doesn't give a damn) there's no difference between pushing yourself off of something or pushing it away, either of them makes the two of you move appart. What the rocket will do is push gas away very very fast, or push itself away from the gas pretty fast.
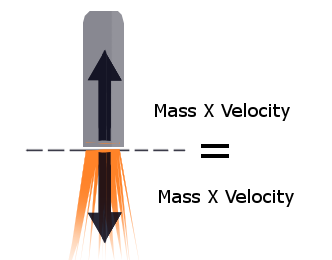
Why are we pushing air?
While in principle we could fling metal goons out the back at lethal velocities, this will make us fail our second goal. Also it's impractical and I have not yet been able to train my pet rat to roll over, nevermind get it to fastball BB pellets at 11300 m/s.
Even though a gas may not weigh much, we can make it leave the rocket at twice the speed of sound so we still get good push.
How will we make fast gas? By exploding sugar.
The basic principle behind the exploding sugar is one of springy tension energy. Imagine the sugar is made of magnetic balls that are separated by balancing them on sticks between them. If you bump the stick hard enough, the balls will fly towards each other and the mass of balls will jump and spin like the picture below shows:
Note the red arrows show where they are being pulled to, and how the mass of balls fly off like a mouse trap woken from its tensioned slumber.

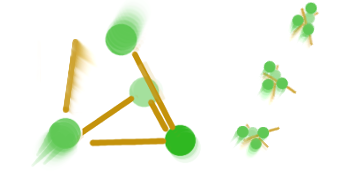
Now imagine we have a container full of these balanced but potentially very energetic lumps of stick magnets. As soon as one is bumped hard enough, it flies off in a direction most likely hitting another another one which hasn't been set off yet. The newly hit one also springs awake and flies off disturbing its neighbors. We end up with a cascade of jumping, flying, spinning lumps hitting the inside of the container and each other with the chaos and might only offered by the mating rituals of covalent and ionic bonding. What we've described here in extreme crudity is what happens when something burns.
This is not a discription you should read to a chemical engineer or a molecular physisist in fear of homicide by braing hemourage.
Organizing the chaos
So now we have a container full of energetic bumpy things, how do we get them all to push in the same direction so we can move the container? First let's imagine what will happen if we just make a hole on the one side. Since all the molecules are bouncing around in random directions, some of them will fly out of this opening, and others will hit the top end of the container. But there's a lot of push that goes missing if you just let them fly out whichever way they'd like. Look at the following diagram:

The above diagram shows that we'd expect if the particles didn't bump into each other, but only bounced along the walls. This fails to take into account that they will still be colliding with each other as they come out of the opening, causing some of them to bounce agains the walls around the container like the picture below shows:
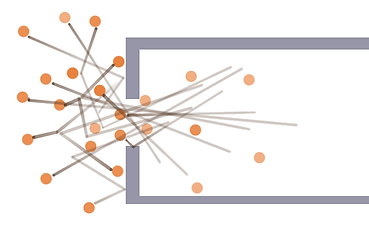
Even though we now have some particles bouncing against the top of the container, and some bouncing against the bottom outside the opening, notice how many fly off at skew angles. Maybe the last time one skew flying one hit the container it was against one of the side walls, pushing the container sideways untill some other particle bounced off the other side and countered the effect. That's a lot of pushy potential that's gone to waste because they didn't bounce off in the right direction if you know what I mean right? If we get them all to fly off straight to the back, they will push the container straight forward with no counterproductive and wasteful side bouncing.
Introducing the Nozzle:
A nozzle is a cleverly shaped opening that makes anything that is blown through it go striaght out the back, no side bouncing. To try and show what I mean, imagine we could pause the world right as the angry mob of bouncing wriggeling things went through the opening:

Right before the particles explode out the hole, notice the arrows that shows in which direction they're about to fly off to.
Imagine we can put a plate in the way of each of those sideways flying particles and bounce them straight backwards.
The picture below shows this:
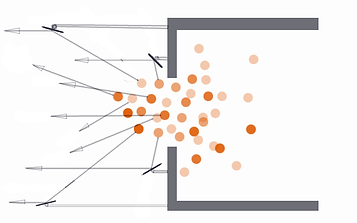
Even though not all of them were caught and redirected, we stopped some of the really bad ones and gained a lot of foreward push that would have been wasted on sideways push!
Since we can't predict or catch every single particle of gas that will fly out the hole and redirect it, we can see a pattern in the angles of the plates and make something that will do the same job, but that's easier to build:
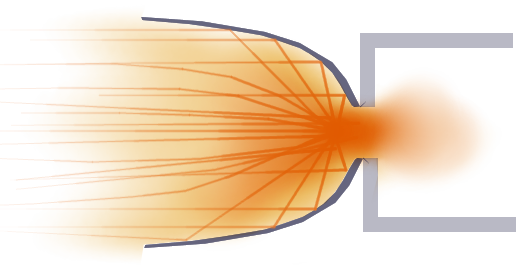
Even though getting the particles to bounce straight back isn't the only reason for the bell like shape of a rocket nozzle, this should give you a better idea of how important the nozzle is in getting all the speed energy from the gas to go into speed energy for the rocket.
Here's another very important thing to understand about the nozzle, it will require you to keep two things in mind:
1) As temperature goes up, pressure goes up
If you realize that bouncy, fast, and angry particles of gas push harder outwards than cold, slow and calm particles (in otherwards their pressure goes up with their tempreture [or temperment if we stick to the personifications] ). Then, if you had the same amount of hot and energetic gas particles on one side of a room as cool and cilled out particles on another, the hot energetic mob would squeeze and push the slow cold ones out of the way.
2) When energy is taken from the speedy particles, they slow down and pressure drops.
Slowing down is the same as getting colder. When the particles collide with something they can push away, they spend some of the energy they had in speeding the thing they bounced into up, and therefore they slow down themselves. Like when you hit one pool ball into another one, the first one practically stops, having given all its energy to the one that speeds away.
This means everytime a particle hits the nozzle and bounces backwards, it slows down a little becasue it sped up the casing and nozzle by pushing against it.
With these two concepts, we can see how the pressure and temperature of the gas decreases as it flows down the nozzle. Remember that the air in the atmosphere is also made of particles that bounce and jiggle like the ones we made inside the rocket casing, even though they're much more relaxed and cooled down.
Now you have to ask what happens when:
a) The gas pressure is higher than the atmosphere pressure:
b) The gas pressure is lower than the atmosphere pressure
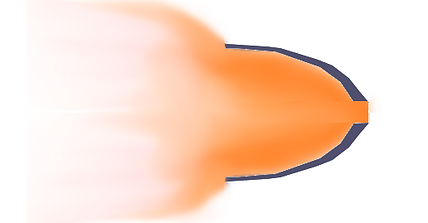
Here we can see if the gas that comes out the back of the nozzle is still at a higher pressure than the atmosphere gas, it will suddenly expand untill it's the same pressure and there's a lot of good push that goes missing. This is exactly the same situation we had when we just made a opening in the back of the container and the gas could fly off in all directions. It is still better than just a hole, because we did recover a lot of sideways push from the chaotic bouncers.
In this case the nozzle should have been made larger, or had a slightly different shape so that the final pressure of the gas is the same as the atmosphere.
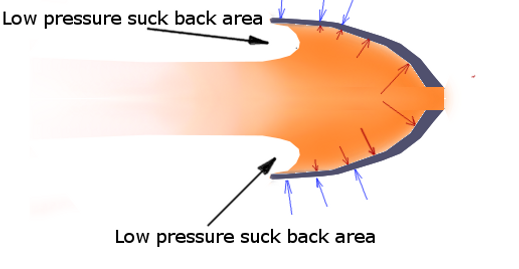
In this case, the nozzel expands the gas so much that the pressure drops below that of the atmosphere. To understand why this is bad, realize that the atmosphere particles all bounce agains the outside of the nozzle and actually push the rocket in the opposite direction. The blue arrows show the atmosphere pishing, and the red arrows show the rocket gas pushing. You can see how the red arrows decrease as the pressure drops.
If the gas inside the nozzle isn't at a higher pressure, they won't win the push-of-war fight and the rocket will be sucked back by the low pressure regions indicated here.
In this case the nozzle should have been made shorter or narrower so that the gas ends at atmospheric pressure.
Just on an interesting side note, if the section above made sense to you, think about how a rocket gets affected by the decreasing pressure in the atmosphere as its altitude increases. Look at these two pictures as a hint:

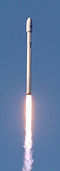
SpaceX Falcon 9 at sea Level
SpaceX Falcon 9 high altitude
Just on an interesting side note, if the section above made sense to you, think about how a rocket gets affected by the decreasing pressure in the atmosphere as its altitude increases. Look at these two pictures as a hint:
Making the rocket fuel (AKA Explodable sugar)
Here I will be a bit more specific as to what will happen to the sugar. Sugar consists of oxygen, carbon, and hydrogen particles. The amount of each or how they stick together to make sugar is not important here. Instead, what I want to focus on is where all that jumpy spring energy is stored that we will release.
You can imagine when the chaos of unbalancing the balls and sticks structures end, most of the balls will be closer together or touching each other than when they first started. Their stored energy has gone into flinging them all over the place. But it's not really little sticks keeping them apart at the beginning, it's other balls. The're placed together in such a way that they're not as close to each other as they could be, and bumping the arangement hard enough sends the molecules crashing into and sticking to molecules which will allow them to be closest to one another.
Like we said before, this whole process is known as burning, and simply put, the sugar has all its molecules further stretched apart from each other than they will be when it burns. After being burned, they get to pair up with each other into groups of water and carbon dioxide which is the closest they can stick to other molecules.
You break up the sugar molecule which takes a little work, but when you bring the parts back together they will fly at each other into their burnt configuration with more force than it took to pull them apart, therefore releasing their stored spring energy.
Why burn sugar?
It sounds strange to someone who usually associates it with chocolates and coke. But food is where we get our energy from, and sugar is a food with
one of the highest energy contents (most of the time people can't even use all the energy the sugar has and their body then stores the rest as fat!).
Where do we get oxygen to burn the sugar if it's sealed in a container?
To burn the sugar, we need to give it some extra oxygen. There isn't enough to go around from just the sugar itself, and the molecules won't be able to get rid of all their energy and settle into a lower energy state if they don't all get the oxygen they need to pair with. Besides the fact that the sugar will be sealed inside the rocket and we can't get air to it, we won't ever be able to pump enough air into the container to enable the sugar to explode instead of burn like a candle anyway.
To produce all the oxygen for our combustion needs we will need the help of another chemical called an oxidizer. It's simply a molecule like the sugar, but when it gets heated ( bumping it so hard that it goes unstable) it breaks up into a lot of oxygen and some other molecules. So, basically, heat it up, and it gives you oxygen.
Burn rate:
Remember how we said the sugar molecules will start reacting like a room full of mouse traps where ones that spring open will hit others and make them spring open too? Imagine we had a very long narrow hall full of mouse traps, or those magnet balls and sticks. If we triggered the one side, we would see a wave of propagating chaos move down the hall as the traps or magnet balls trigger each other. Like a mexican wave of woken up spring energy. If we could measure how far that wave travelled per second, then you'd agree we'd be able to know how many of those energetic bouncing hot particles was released every second.

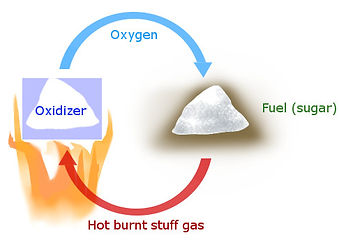
This wave of breaking up and flying off particles can be slow, as in the case of a paper burning, or fast like a match head. If you can control how fast it burns, and control how much burns at the same time, then you can control how much hot speedy gas you make, and therefore control how much push your rocket gets.
Since I decided to use sugar as my fuel and saltpeter for my oxidizer (saltpeter is the same oxidizer that blackpowder uses. It's probably the most commonly known one) our reaction rate is pretty much set by their chemical properties. A more unstable fuel, or unstable oxidizer may give us a faster burn, but we don't necessarily want it to burn too fast.
If we produce too much gas at a time, it may not be able to get out the nozzle fast enough and cause the rocket to become over pressurized and explode. Even if the rocket could handle the pressure, we mentioned that we want the final pressure of the gas to be that of the atmosphere, so we may just be wasting good push if too much fuel burns at a time.
Predicting how high it will go
There's an interesting thing to note about rockets, and it's that they get lighter and lighter as they burn and push away their fuel. Think about it, you start off with most of the rocket filled with whatever you're going to turn into hot gas, and as it burns, you blow it out the back. So here's a little graph to show what happens to a rocket that, say, weighs half a kilogram and has 1kg of fuel which it burns at 0.1 kg per second:

The rocket starts off at a total mass of 1.5kg, and since we burn and blow out fuel at 0.1kg per second, it takes 10 seconds for us to use all the fuel. At the end of the burn, all that is left is the 0.5kg casing of the rocket (hopefully going very fast after it pushed all that other mass away).
If you understand what's happening above, then you should think about what will happen to the acceleration (how fast the rocket picks up speed) . You'd agree that you can make a light object go faster than a heavy object when you push just as hard on both right? We also burn the same amount of fuel through the same nozzel untill all the fuel is gone, so the amount of push stays the same. But the rocket gets lighter and lighter, so in the beginning the amount of push from the nozzle will make it pick up a little bit of speed at a time, but by the end of the burn, the rocket will be able to pick up a bunch of speed quickly.
Here's another graph that will show how the Mass, acceleration, and speed are related:
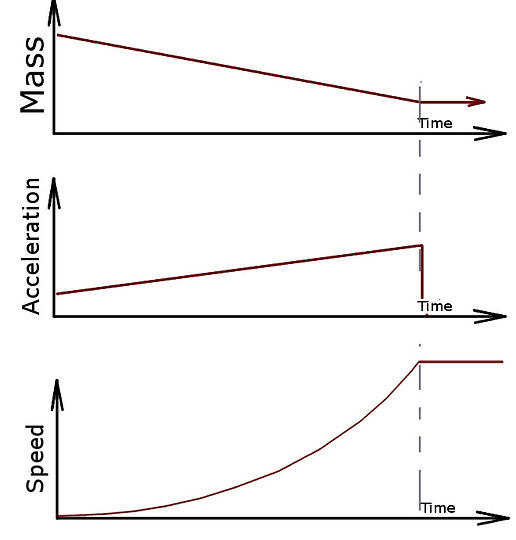
Those of you who know something about integration, or if you're just good at playing with quantities in your head, you'll realize the area under the line of the acceleration up to a certain time on the graph is equal to the speed at that time.
To do the energy release calculations we have to take all the molecules that play a role into account. And it may seem a bit complicated because we have to deal with the saltpeter as well which is made of potassium, nitrogen and oxygen. But having different molecules is only like having different types of elastic bands, everything still adds up but you just have to do a bit more sums.
When we start, we have sugar and saltpeter. When we end, we have water, carbon dioxide, nitrogen and salt
The salt is just made by the left over potassium and some carbon and oxygen, don't worry about it, it's just like any other molecule and it also has an enthalpy of creation so all that complexity is summed up into one number that can be added and subtracted.
So: (ENRERGY OUT)heat = (ENERGY STORED)start - (ENERGY STORED)end
Below I drew a picture of the reactants (the things that will react and burn) and the products (what they become afterwards)
Notice that for every molecule in the mixture to be able to react and release its energy, there needs to be an ideal mixture of sugar to saltpeter. How much of each molecule you need I already worked out and it's not important for understanding what's going on but if your interested in how to do this it's called stoichiometry.
5(sugar) + 48(Saltpeter) => 24(Salt) + 36(Carbon dioxide) + 55(Water) + 24(Nitrogen)
Reactants

Reactants with energy to create their structure underlined in red (enthalpy of creation).
I got all my chemical properties like enthalpy of formation and specific heats from the NIST website here: http://webbook.nist.gov/
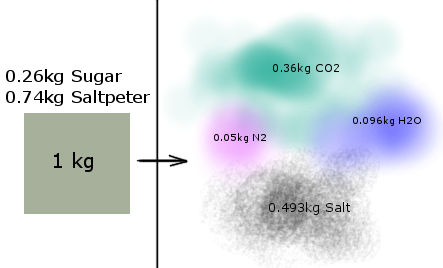
Richard Nakka did some amazing work in calculating all these values, he even added some really cool graphs that show the velocity, pressure, and thrust ratio through the engine. You should check it out here:
​
Richard Nakka's engine calculations
What has t be noted here is that is the absolute ideal reaction and circumstances for that reaction. In reality, not all the molecules of sugar will be broken down or react with the other molecules they should. Some molecules won't hook up to their lowes possible enrgy state and form weird complex molecules that took some of the enrgy away. The whole burning thing is absolute chaos and uncontrollable and dirty.
Next we will work out the maximum expected pressure that will develop from those exit gasses and temperatures. Like we said before, when temperature goes up, pressure will go up. Pressure will also obviously go up with more gass particles squeezed into the same space. I will use the ideal gas law to tell me the pressure. Again taking the weighted average of the gas constants of the various exit gasses:
So now we know that we're dealing with hot gasses and high pressures. We need to find materials and parts that can handle these violent conditions. But exactly how much pressure should they handle? How much temperature do they have to stand? While these two questions are very important, a factor that may make the answer to them much less dramatic is time. If we ask "how much pressure for how long" and "how much heat for how long" the answers can be drastically different. If you Frisbee throw a piece of bread through a bonfire's flames you don't get toast, maybe slightly seared edges at the most.
So can we find things in my kitchen and backyard that will stand rocket fire explosions? Most likely, to be sure we need math, so let's make some.
​
WARNING The following section I will put in blue contains some weird math and incomplete and complex explanations of thermodynamic concepts (If that word scared you, then this warning probably applies to you). It's not necessary to understand the stuff in blue, but it's here for those interested in seeing all my steps.
First, what tempretures (remember this is just fancy for how fast the woken up partcles jump around) will a sugar and saltper mixure get to?
To work this out, we need two things:
1) How much energy is released when we burn an ideal sugar and saltpeter mixture?
2) How much does the temperature rise for every bit of energy we release?
To answer the first question, we can act like the bonds between the particles act almost like a stretched bungee cord. When the molecules are in the sugar structure they are stretched far appart and there's energy stored in the bonds like there would be in the elastic of the bungee chord. To release the energy from the chord you have to stretch it a little to un-hook it (add a bit of energy), then you can let it go and it will spring down to the length of the new shorter length. The energy you released has gone into shooting off the particles like a rubberband gun.

Same as releasing the stored energy in a bit of sugar by breaking it into carbon dioxide and water, we can add energy to carbon dioxide and water to make sugar again (just like when you stretch the chords and hook them on). In fact, this is what plants do with photosynthesis. They take some carbon dioxide, and water and use a bit of energy from the sun to stretch the molecules apart and place them together carefully so they don't snap back down. The energy it takes to create a molecule of something is called the enthalpy of creation. It's also easy to see that the energy we will get out is the difference between the two energy states.
Before we talk about the energy and heat and stuff involved with sugar and saltpeter I think a simpler example should be given. We will use oxygen and hydrogen, the same chemicals the space shuttle uses.
Products and the energy to create their structure
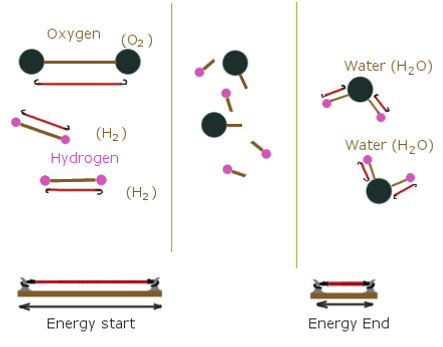
The angry high speed particles that jump around and push against the rocket nozzle is the water particles. That white trail behind the space shuttle is steam. Normal water steam that you see coming out of your kettle.